Introduction to Back Work Ratio
The back work ratio is a crucial parameter in the field of thermodynamics, specifically in the study of gas and steam turbines. It provides valuable insights into the efficiency and performance of these machines. In this section, we will delve into the definition of the back work ratio and explore the formula used to calculate it.
Definition of Back Work Ratio
The back work ratio is a measure of the amount of work required to operate a turbine compared to the work it produces. It is expressed as a ratio and is an essential factor in determining the overall efficiency of a turbine.
In a gas or steam turbine, the back work ratio represents the portion of the work output that is used to drive the compressor or pump. This work is necessary to maintain the required pressure ratio and ensure the continuous operation of the turbine.
A high back work ratio indicates that a significant portion of the turbine’s power output is used to drive the compressor or pump, resulting in lower overall efficiency. Conversely, a low back work ratio signifies that a smaller proportion of the power output is consumed by these components, leading to higher efficiency.
Formula for Back Work Ratio
The back work ratio can be calculated using the following formula:
Back Work Ratio = (Work Input - Work Output) / Work Output
To understand this formula better, let’s break it down:
-
Work Input: This refers to the amount of work required to operate the turbine. It includes the work needed to drive the compressor or pump, as well as any other external work input.
-
Work Output: This represents the actual work produced by the turbine. It is the net power output available for useful work, such as generating electricity or driving machinery.
By subtracting the work output from the work input and dividing it by the work output, we obtain the back work ratio. This ratio provides a quantitative measure of the energy losses within the turbine system.
It is important to note that the back work ratio is influenced by various factors, including turbine efficiency, compressor efficiency, pressure ratio, and isentropic efficiency. These parameters play a significant role in determining the overall performance and effectiveness of the thermodynamic cycle.
In the next sections, we will explore each of these factors in more detail and understand their impact on the back work ratio.
Back Work Ratio in Gas Turbine Engines
The back work ratio is an important parameter that measures the efficiency of a gas turbine engine. It represents the amount of work required to drive the compressor compared to the work produced by the turbine. In other words, it quantifies the energy losses within the engine.
Reasons for Relatively High Back Work Ratio
There are several factors that contribute to a relatively high back work ratio in gas turbine engines. Understanding these reasons is crucial for optimizing the performance of these engines.
-
Compressor Efficiency: The efficiency of the compressor plays a significant role in determining the back work ratio. A less efficient compressor requires more work to achieve the desired pressure ratio, resulting in a higher back work ratio.
-
Pressure Ratio: The pressure ratio, which is the ratio of the compressor outlet pressure to the inlet pressure, also affects the back work ratio. Higher pressure ratios generally lead to higher back work ratios.
-
Isentropic Efficiency: The isentropic efficiency of the compressor and turbine stages impacts the back work ratio. Lower isentropic efficiencies result in higher back work ratios, as more work is required to compensate for the energy losses.
Typical Back Work Ratio Values for Gas Turbine Engines
The back work ratio values for gas turbine engines can vary depending on various factors such as engine design, operating conditions, and specific applications. However, there are some typical ranges that can be observed.
-
Aircraft Gas Turbines: In aircraft gas turbines, the back work ratio is usually in the range of 0.3 to 0.5. These engines are designed to prioritize power output and fuel efficiency, which leads to relatively lower back work ratios.
-
Industrial Gas Turbines: Industrial gas turbines, used in power generation and other industrial applications, tend to have higher back work ratios. Typical values for these engines range from 0.5 to 0.8. The higher back work ratios are often a result of the need for higher power output and efficiency.
-
Combined Cycle Power Plants: Gas turbines used in combined cycle power plants, where the exhaust gases are used to generate steam for a steam turbine, have lower back work ratios compared to standalone gas turbines. The back work ratio for these systems can range from 0.2 to 0.4.
It’s important to note that these values are general guidelines and can vary depending on specific engine configurations and operating conditions.
In conclusion, the back work ratio is a critical parameter in gas turbine engines that measures the efficiency of the engine. Understanding the reasons for a relatively high back work ratio and typical values for different types of gas turbines can help engineers and operators optimize the performance of these engines. By focusing on improving compressor efficiency, pressure ratio, and isentropic efficiency, it is possible to reduce the back work ratio and enhance the overall efficiency of gas turbine engines.
Back Work Ratio in Brayton Cycle
The back work ratio is an important parameter in the Brayton cycle, which is a thermodynamic cycle commonly used in gas turbine engines. It quantifies the amount of work required to drive the compressor compared to the work output of the turbine. In this section, we will explore the explanation of the Brayton cycle and the formula used to calculate the back work ratio.
Explanation of Brayton Cycle
The Brayton cycle is a thermodynamic cycle that describes the operation of a gas turbine engine. It consists of four main processes: compression, combustion, expansion, and exhaust. Let’s take a closer look at each of these processes:
-
Compression: In this process, the air is drawn into the compressor and compressed to a higher pressure. The compressor plays a crucial role in increasing the pressure of the air before it enters the combustion chamber.
-
Combustion: Once the air is compressed, it is mixed with fuel and ignited in the combustion chamber. The combustion process releases a large amount of heat, which increases the temperature and pressure of the working fluid.
-
Expansion: The high-pressure, high-temperature gas from the combustion chamber is expanded in the turbine. As the gas expands, it loses energy, which is converted into mechanical work to drive the turbine and any attached load, such as an aircraft engine or a power generator.
-
Exhaust: After the expansion process, the gas is exhausted from the turbine. It may still contain some energy, but it is typically at a lower pressure and temperature compared to the gas entering the turbine.
The Brayton cycle is often referred to as an ideal cycle, assuming certain ideal conditions such as no losses due to friction or heat transfer. However, in real-world applications, these losses are inevitable and can impact the overall efficiency of the cycle.
Back Work Ratio Formula in Brayton Cycle
The back work ratio (BWR) is defined as the ratio of the work required to drive the compressor to the work output of the turbine. It is an essential parameter in determining the overall efficiency of the gas turbine engine. The formula to calculate the back work ratio is as follows:
BWR = (Work Input to Compressor) / (Work Output of Turbine)
The work input to the compressor is the energy required to compress the air, while the work output of the turbine is the energy produced by the expansion of the high-pressure gas. By comparing these two values, we can determine the efficiency of the Brayton cycle.
A high back work ratio indicates that a significant portion of the turbine’s output work is used to drive the compressor, resulting in lower net power output. Conversely, a low back work ratio implies that the turbine is more efficient, as less work is required to drive the compressor.
It is important to note that the back work ratio is influenced by various factors, including the efficiency of the compressor and turbine, the pressure ratio across the compressor and turbine, and the isentropic efficiencies of these components. Optimizing these factors can help improve the overall efficiency of the Brayton cycle.
In conclusion, the back work ratio is a crucial parameter in the Brayton cycle, as it quantifies the efficiency of the gas turbine engine. By understanding the explanation of the Brayton cycle and the formula to calculate the back work ratio, engineers and designers can make informed decisions to optimize the performance of gas turbine systems.
Back Work Ratio in Rankine Cycle
Explanation of Rankine Cycle
The Rankine Cycle is a thermodynamic cycle commonly used in power plants to generate electricity. It is a closed-loop cycle that utilizes both a heat source and a heat sink to convert heat energy into mechanical work. The cycle consists of four main components: a boiler, a turbine, a condenser, and a pump.
The process begins in the boiler, where heat is added to the working fluid, typically water, to convert it into high-pressure steam. This high-pressure steam then enters the turbine, where it expands and does work by driving the turbine blades. As the steam expands, its pressure and temperature decrease.
After leaving the turbine, the low-pressure steam enters the condenser, where it is cooled and condensed back into liquid form. This condensation process releases heat, which is transferred to a cooling medium, such as water from a nearby river or ocean. The condensed liquid is then pumped back to the boiler to repeat the cycle.
Back Work Ratio Formula in Rankine Cycle
The back work ratio (BWR) is a parameter used to evaluate the performance of a Rankine Cycle. It represents the ratio of the work required to operate the pump to the net work output of the turbine. Mathematically, it can be expressed as:
BWR = (Work input to pump) / (Net work output of turbine)
The work input to the pump is the energy required to increase the pressure of the working fluid from the condenser pressure to the boiler pressure. This work input is typically expressed in terms of kilojoules per kilogram (kJ/kg) of the working fluid.
On the other hand, the net work output of the turbine is the difference between the work done by the turbine and the work done by the pump. The work done by the turbine is the energy extracted from the steam as it expands in the turbine, while the work done by the pump is the energy required to increase the pressure of the working fluid.
The back work ratio is an important parameter because it indicates the efficiency of the Rankine Cycle. A lower back work ratio implies a more efficient cycle, as less work is required to operate the pump relative to the work output of the turbine. Conversely, a higher back work ratio indicates a less efficient cycle, as more work is needed to operate the pump.
In practice, engineers strive to minimize the back work ratio by optimizing the design and operation of the Rankine Cycle. This can be achieved by using efficient pumps and turbines, maximizing the temperature difference between the heat source and heat sink, and reducing losses due to friction and heat transfer.
By carefully considering the back work ratio, engineers can improve the overall efficiency and performance of power plants that utilize the Rankine Cycle. This, in turn, leads to reduced energy consumption and lower environmental impact.
Back Work Ratio in Otto Cycle
The back work ratio is an important parameter in the Otto cycle, which is a thermodynamic cycle commonly used in internal combustion engines. It helps us understand the efficiency of the cycle and the amount of work required to operate the engine. In this section, we will explain the Otto cycle and discuss the formula for calculating the back work ratio.
Explanation of Otto Cycle
The Otto cycle is a theoretical thermodynamic cycle that describes the operation of a typical gasoline engine. It consists of four processes: intake, compression, combustion, and exhaust. During the intake process, the fuel-air mixture is drawn into the cylinder. In the compression process, the mixture is compressed to increase its temperature and pressure. The combustion process involves the ignition of the compressed mixture, resulting in a rapid expansion of gases and the generation of power. Finally, in the exhaust process, the burned gases are expelled from the cylinder.
The Otto cycle is an idealized representation of the actual engine operation, assuming certain ideal conditions such as perfect combustion, no heat loss, and ideal gas behavior. Despite these simplifications, the Otto cycle provides a useful framework for analyzing engine performance.
Back Work Ratio Formula in Otto Cycle
The back work ratio (BWR) is defined as the ratio of the work required to operate the engine’s auxiliaries (such as the compressor and the pump) to the net work output of the engine. It is an indicator of the efficiency of the cycle and is typically expressed as a percentage.
The formula for calculating the back work ratio in the Otto cycle is:
BWR = (Work Input to Compressor + Work Input to Pump) / Work Output of Engine * 100
The work input to the compressor is the work required to compress the air-fuel mixture during the compression process. It is influenced by factors such as the compression ratio and the efficiency of the compressor. The work input to the pump is the work required to circulate the coolant or lubricant in the engine. It depends on the flow rate and the pressure difference across the pump.
The work output of the engine is the net work produced during the power stroke of the combustion process. It is influenced by factors such as the pressure ratio, the isentropic efficiency of the combustion process, and the mechanical efficiency of the engine.
By calculating the back work ratio, engineers can assess the efficiency of the engine’s auxiliaries and identify areas for improvement. A high back work ratio indicates that a significant portion of the engine’s power is consumed by the auxiliaries, reducing the overall efficiency of the system. On the other hand, a low back work ratio suggests that the auxiliaries are operating efficiently, allowing more power to be delivered to the output.
In conclusion, the back work ratio is an important parameter in the Otto cycle, providing insights into the efficiency of the engine’s auxiliaries. By understanding and optimizing the back work ratio, engineers can enhance the overall performance of internal combustion engines.
Significance of Back Work Ratio
The back work ratio is an important parameter in the field of thermodynamics, specifically in the study of gas turbines and steam turbines. It plays a crucial role in determining the overall efficiency and performance of these power generation systems. In this section, we will explore the importance of the back work ratio and how it is calculated.
Importance of Back Work Ratio
The back work ratio is a measure of the energy required to drive the compressor or the pump in a thermodynamic cycle. It represents the fraction of the work output that is used to overcome the losses in the turbine or the compressor. A low back work ratio indicates that a significant portion of the work output is consumed by these losses, resulting in reduced overall efficiency.
One of the key reasons why the back work ratio is significant is its direct impact on the efficiency of the turbine. The back work ratio affects both the turbine efficiency and the overall power output of the system. A higher back work ratio means that more energy is required to drive the compressor or the pump, resulting in a decrease in the net power output of the turbine.
Additionally, the back work ratio also influences the pressure ratio and the isentropic efficiency of the compressor or the pump. These parameters are crucial in determining the performance of the entire thermodynamic cycle. A higher back work ratio leads to an increase in the pressure ratio, which can have a positive effect on the overall efficiency of the system.
Furthermore, the back work ratio is closely related to the efficiency of the turbine. By minimizing the losses in the turbine or the compressor, the back work ratio can significantly improve the overall efficiency of the system. This is particularly important in power generation applications, where even a small increase in efficiency can result in substantial cost savings and environmental benefits.
Calculation of Back Work Ratio
The back work ratio can be calculated using the following formula:
Back Work Ratio = (Work Input - Work Output) / Work Output
To calculate the back work ratio, we need to determine the work input and the work output of the system. The work input represents the energy required to drive the compressor or the pump, while the work output represents the useful work produced by the turbine.
In a gas turbine, the work input is typically calculated by measuring the power input to the compressor, while the work output is determined by measuring the power output from the turbine. Similarly, in a steam turbine, the work input is calculated based on the enthalpy change of the steam, while the work output is determined by measuring the power output from the turbine.
Once we have the values for the work input and the work output, we can substitute them into the formula to calculate the back work ratio. The resulting value provides us with a quantitative measure of the energy losses in the turbine or the compressor.
In conclusion, the back work ratio is a significant parameter in the study of gas turbines and steam turbines. It directly influences the efficiency and performance of these power generation systems. By understanding the importance of the back work ratio and how to calculate it, engineers and researchers can optimize the design and operation of turbines to achieve higher efficiency and improved performance.
Conclusion
In conclusion, the back work ratio is a crucial metric that helps measure the efficiency of a heat engine or a refrigeration system. It indicates the amount of useful work output obtained from a system compared to the amount of work input required to operate it. A higher back work ratio signifies a more efficient system, as it indicates that a larger proportion of the input energy is converted into useful work. On the other hand, a lower back work ratio suggests that a significant portion of the input energy is lost as waste or used to operate auxiliary components. By optimizing the back work ratio, engineers and designers can improve the overall performance and energy efficiency of various systems, including power plants, engines, and refrigeration units. It is important to consider the back work ratio when evaluating and comparing different systems, as it provides valuable insights into their energy conversion capabilities. By understanding and optimizing the back work ratio, we can strive towards more sustainable and energy-efficient technologies that minimize waste and maximize the utilization of available resources.
Frequently Asked Questions
Q: Why are the back work ratio relatively high in gas turbine engines?
A: Gas turbine engines have relatively high back work ratios because a significant portion of the work produced by the turbine is used to drive the compressor, resulting in a higher energy requirement for the overall operation of the engine.
Q: What are typical back work ratio values for gas-turbine engines?
A: Typical back work ratio values for gas turbine engines can vary depending on the specific design and operating conditions. However, values between 0.3 and 0.5 are commonly observed in practice.
Q: What is back work ratio?
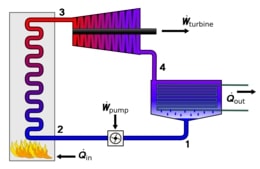
A: Back work ratio is a thermodynamic parameter that represents the ratio of work required to drive the compressor to the work produced by the turbine in a thermodynamic cycle. It is an indicator of the efficiency of the overall cycle.
Q: What is the back work ratio of this cycle?
A: The back work ratio of a specific cycle depends on the design and operating conditions of the system. It can be calculated by dividing the work required to drive the compressor by the work produced by the turbine.
Q: What is back work ratio in Brayton cycle?
A: In the Brayton cycle, the back work ratio represents the ratio of work required to drive the compressor to the work produced by the turbine. It is an important parameter that affects the overall efficiency of the cycle.
Q: What is back work ratio formula?
A: The formula to calculate the back work ratio is: Back Work Ratio = Work Required to Drive Compressor / Work Produced by Turbine.
Q: What is back work ratio of gas turbine?
A: The back work ratio of a gas turbine represents the ratio of work required to drive the compressor to the work produced by the turbine. It is an important parameter that affects the efficiency and performance of the gas turbine.
Q: What is back work ratio in thermodynamics?
A: In thermodynamics, back work ratio is a parameter that measures the efficiency of a thermodynamic cycle. It represents the ratio of work required to drive the compressor to the work produced by the turbine.
Q: What is turbine efficiency?
A: Turbine efficiency is a measure of how effectively a turbine converts the energy of a fluid (such as gas or steam) into mechanical work. It is typically expressed as a percentage and is influenced by factors such as design, operating conditions, and losses.
Q: What is compressor efficiency?
A: Compressor efficiency is a measure of how effectively a compressor increases the pressure of a fluid. It is typically expressed as a percentage and is influenced by factors such as design, operating conditions, and losses.
Hi ….I am Abhishek Khambhata, have pursued B. Tech in Mechanical Engineering. Throughout four years of my engineering, I have designed and flown unmanned aerial vehicles. My forte is fluid mechanics and thermal engineering. My fourth-year project was based on the performance enhancement of unmanned aerial vehicles using solar technology. I would like to connect with like-minded people.